EMITTANCE DILUTION DUE TO SLOW ALIGNMENT DRIFTS IN THE MAIN LINACS OF
THE NLC*
R. Assmann, C. Adolphsen, K. Bane, T.O. Raubenheimer, K. Thompson
Stanford Linear Accelerator Center, Stanford, CA 94309, USA
Abstract
The tight tolerances in the main linacs of the Next Linear
Collider (NLC) result in a large sensitivity of the beam
emittance to slow alignment drifts. Once the accelerator is
tuned, the optimized emittances must be maintained. Slow
alignment drifts will make resteering and reoptimization
necessary. The frequency of these linac reoptimizations is an
important parameter that determines how well the linear
collider can be operated. We present simulation results that
address this question for the main linacs of the NLC. We will
show that the effects of alignment drifts can indeed be
handled.
Introduction
The alignment stability in the main linacs of NLC
determines how often the alignment and correction
algorithms need to be applied. It has been shown that static
imperfections in NLC can be corrected down to the required
levels [1]. Linac stability effects are dominated by quadrupole
alignment drifts; the quadrupoles generally have the tightest
alignment tolerances. Here, we do not discuss the BPM
stability. However, the requirements are tight. For the
alignment algorithm we require a 2 µm static rms offset
between the BPM and quadrupole centers [1]. This tolerance
can be achieved with a time consuming beam-based
alignment procedure and it must be stable over significant
periods of times (days). The question of BPM stability is
discussed in [2]. Since quadrupoles and BPM's are
mechanically mounted together, the BPM stability that can be
achieved is mainly determined by the BPM electronics, cables
and similar factors. Results from beam-based alignment
experiments at HERA and LEP show that the BPM to
quadrupole alignment presently is being done with an
absolute accuracy of better than 10 µm [3, 4]. The results in
HERA are found to be remarkably stable over years.
Simulation parameters
The simulations were done with the computer program
LIAR [5] using the 500 GeV version of NLC-II as defined in
[2]. We consider a single bunch with with a population of
1.1
1010 particles. The injection energy is 10 GeV, the
bunch length is 150 µm, and we include an initial
uncorrelated energy spread of 1.5%. The initial horizontal
and vertical beam emittances are
m-rad and
m-rad. We assume that the chicanes in the
diagnostics stations are switched off. The simulations were
done for the vertical plane where the small initial emittance
makes it much harder to avoid emittance dilutions. A realistic
BNS damping setup is included [2].
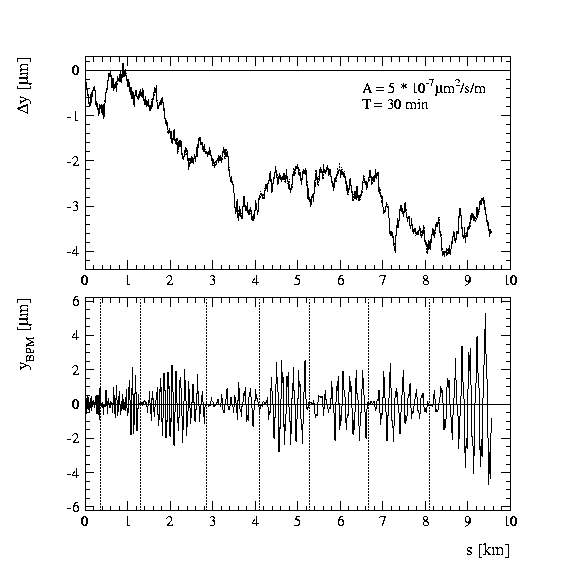
Figure 1: Example of ATL-like alignment drifts. The upper
plot shows the displacements of quadrupoles, RF-structures
and BPM's after 30 minutes with an A-coefficient of 5
10-7
µm2/sec/m. The alignment was flat initially. The lower plot
shows the corresponding trajectory offsets yBPM at the BPM's.
The dotted lines indicate the locations of trajectory feedbacks
where y and y' are corrected back to zero. Thus the size of
coherent betatron oscillations is constrained.
ATL-like alignment drifts
In order to model alignment drifts, we use the ATL-
model [6]. This predicts that the rms vertical misalignment
(in µm) deteriorates with time T (in seconds) and over
the length L (in m) as follows:
Recent studies [7] show that a constant A of better than 5
10-7
µm2/sec/m can be measured on the SLAC site. We use
A = 5
10-7
µm2/sec/m.
Figure 1 illustrates ATL-like alignment drifts. It shows the displacements
and corresponding
trajectory offsets ybpm at the BPM's after 30 minutes. The
offsets of quadrupoles, BPM's, and RF-structures are overlaid
in the plot and are essentially indistinguishable. The
trajectory offsets at the BPM's show the coherent betatron
oscillations that build up. The dotted lines indicate the
locations of seven trajectory feedbacks that constrain y and y'
to zero. The coherent betatron oscillations are thus broken up
into eight smaller oscillations. The oscillation amplitude is a
few µm and is large enough to be detected easily with the
NLC single shot BPM resolution of 1 µm.
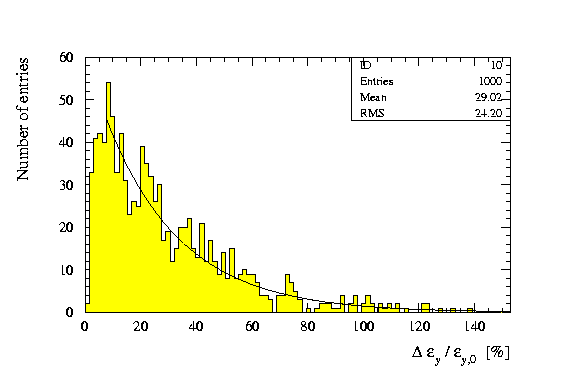
Figure 2: Histogram of vertical emittance growth
for 1000 different error distributions. ATL-like
alignment drifts over 30 minutes with an A of 5
10-7
µm2/sec/m are assumed. The average emittance growth is
29.0%
0.8%.
The solid curve shows an exponential fit for
large emittance dilutions.
A histogram of the vertical single-bunch emittance
growth from alignment drifts after 30 minutes is shown in
Figure 2. The average emittance growth is found to be 29.0%
0.8%.
Note, however, the exponential distribution for large
emittance dilutions. The most probable emittance growth is
only about 10%.
The average emittance growth along the
linac is shown in Figure 3. The locations of the trajectory
feedbacks are clearly seen. As a coherent betatron oscillation
builds up the emittance starts to grow rapidly. The feedbacks
stop the fast growth. More effective feedbacks can be
imagined if the rms y trajectory is minimized up to the next
feedback instead of correcting y and y' to zero locally.
Figure 4 shows the average vertical single-bunch
emittance growth for different BNS configurations. All
previous results were obtained using the standard NLC BNS
configuration which requires an energy overhead of 1.3%.
The standard BNS is optimized for the beam-based alignment
algorithm and uses three different Rf phases along the linac.
Figure 4 shows that BNS configurations using higher energy
overheads reduce the emittance growth from 29% to about
16%. One can therefore imagine to trade alignment
performance against better stability.
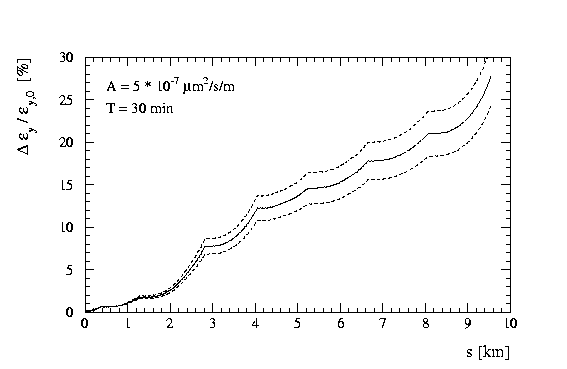
Figure 3: Average vertical emittance growth
along
the linac for ATL-like drifts after 30 minutes. We assume an
A coefficient of 5
10-7 µm2/sec/m. The dashed curves
specify the errorbands around the average (solid curve).
Figure 4: Average vertical emittance growth
from
ATL-like alignment drifts for different BNS configurations.
The BNS configurations are specified in terms of the
associated BNS energy overhead. That is the percentage of the
beam acceleration that is lost due to the Rf phases required for
a given BNS scheme.
Let us relate the ATL-like alignment drifts to the other
results. Since the emittance growth is linear in time we would
get an additional average emittance growth of about 15%
when we assume a beam-based alignment every 30 minutes.
This is about a factor of six smaller than the emittance growth
expected after beam-based alignment of quadrupoles and RF-
structures. It is small enough not to be an important
limitation of the NLC linac performance as long as the linacs
are corrected on an half-hourly to hourly basis. Since the
alignment and correction algorithm does not interfere with
the standard operation, its frequent application should be no
major obstacle.
Conclusion
The dominant stability problem in the NLC main linacs is
caused by drifts of the quadrupole alignment. We simulated
this effect by using the ATL-model with a coefficient
A = 5
10-7 µm2/sec/m as measured at SLAC. We showed
that the alignment drifts drive coherent betatron oscillations
that lead to rapid emittance growth. The addition of seven
trajectory feedbacks breaks the coherent betatron oscillation
into eight smaller oscillations. Assuming a beam-based
quadrupole alignment every 30 minutes, we would expect an
additional average emittance growth contribution of 15%.
Since the emittances roughly add up in quadrature, this is
small compared to the 110% average emittance growth after
beam-based alignment. The alignment algorithm does not
interfere with the normal linac operations, so that it can be
applied very frequently. We conclude that slow alignment
drifts can be handled safely for the NLC linacs, if we assume
a beam line stability similar to or better than the one observed
in the Final Focus Test Beam (FFTB) experiment at SLAC.
References
[1] R. Assmann et al., "Emittance and Trajectory Control in
the Main Linacs of the NLC", these proceedings.
[2] The NLC Design Group, "Zeroth-Order Design Report for
the Next Linear Collider", SLAC report 474 (1996).
[3] M. Böge and R. Brinkmann, "Optimization of Electron
Spin Polarization by Application of a Beam-Based Alignment
Technique in the HERA Electron Ring", proc. of the 4th
IWAA95, Nov. 14 - 17, 1995, KEK, Japan. V 413.
[4] I. Barnett et al., "Dynamic Beam-Based Calibration of
Orbit Monitors at LEP", proc. of the 4th IWAA95, Nov. 14 -
17, 1995, KEK, Japan. V 421.
[5] R. Assmann et al., "LIAR - A Computer Program for
Linear Collider Simulations", SLAC-AP 103 (1996).
[6] V. Shiltsev, "Space-Time Ground Diffusion: The ATL
Law for Accelerators", proc. of the 4th IWAA95, Nov. 14 -17,
1995, KEK, Japan. IV 352.
[7] R. Assmann and C. Montag, "Beamline Stability
Measurements with a Stretched Wire System in the FFTB",
these proceedings.
* Work supported by the Department of Energy, contract DE-AC03-
76SF00515